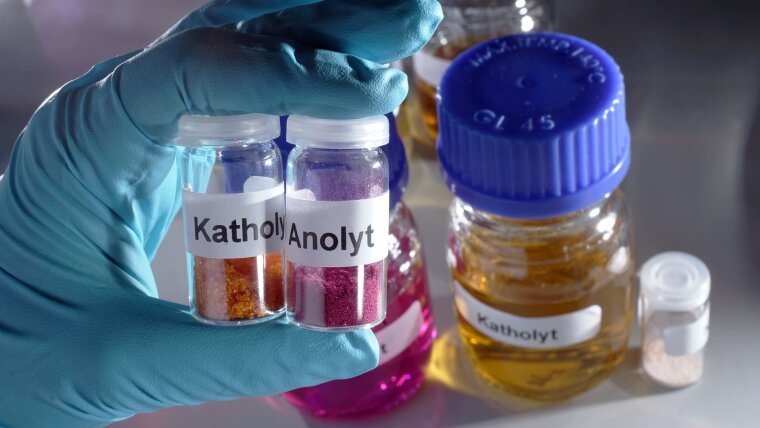
Please note that only the English language projects are listed on this page. A list of all projects can be found here de.
-
Innovation Center CEEC Jena
Funded by the Free State of Thuringia and the European Regional Development Fund (ERDF)
Duration: 2022 to 2023With regard to a sustainable energy supply, energy storage and converters are central components of a successful energy transition. Polymers are promising materials in this context as they allow energy storage (i.e. batteries) and converters (e.g. solar cells) with special properties (e.g. flexible, printable, light weight, low carbon footprint). As part of this project, the infrastructure of the CEEC Jena is to be further expanded with regard to these polymers.
-
Research Unit (FOR) 5301 „FuncHeal“, Project P1: Self-healing electrodes for electro(chemical) energy storage
Prof. Dr. Andrea Balducci, Prof. Dr. Ulrich S. Schubert
Funded by the German Research Foundation
Funding period: 2022 to 2026The self-healing of polymers has been studied intensively in recent years. In doing so, general design principles could be established enabling the preparation of healable polymers. As part of the project, these concepts are now to be transferred to functional materials. Specifically, new types of electrodes for use in organic radical batteries or (super)capacitors are to be developed, which are characterized by their ability to heal themselves.
For this purpose, self-healing polymers with various reversible groups are integrated into the electrode materials in order to ultimately enable cracks and damage to be healed. In addition to the synthesis, a focus will be on the characterization of the materials and, in particular, on the investigation of the self-healing properties. On the one hand, the cracks in the electrodes will be examined optically and tactilely, on the other hand, the restoration of functional properties, such as conductivity or capacity, will be analyzed. In particular, a new method will be established with which the healing of functional materials can be quantified in detail. Finally, the project aims to design new self-healing electrodes for applications in organic radical batteries and supercapacitors and to elucidate structure-property relationships for self-healing functional materials.
-
Research Unit (FOR) 5301 „FuncHeal“, Project P2: Nanostructured block copolymer gel electrolytes based on polyethers
Prof. Dr. Felix H. Schacher
Funded by the German Research Foundation
Funding period: 2022 to 2026The goal of project 2 is to design nanostructured block copolymer gels for use as electrolytes in polymer-based batteries and electric double-layer capacitors.
These materials also feature self-healing properties, whereby the designed nanostructure additionally enables the restoration of the functional properties after mechanical damage. For this purpose, amphiphilic polyether-based ABC triblock terpolymers will be utilized with a (covalently) crosslinkable block A (furfuryl moieties for Diels-Alder reactions or alkynes for copper-catalyzed azide-alkyne chemistry), a block B being soluble in both organic and aqueous electrolytes providing ion mobility (e.g., poly(methyl glycidyl ether)), and a block C carrying charged repeating units for reversible (non-covalent) crosslinks based on combinations of carboxyl and amino moieties or alternatively, zwitterionic comonomers. The synthesis will be accomplished using a combination of sequential living anionic polymerization of the respective glycidyl ether monomers (i.e. furfuryl or alkynyl glycidyl ether for block A, e.g., methyl glycidyl ether for block B, allyl glycidyl ether ether for block C) followed by side chain modification of the C block using thiol-ene click chemistry to realize the charged repeating units.
The characterization will play an important role in this project starting from the comprehensive analysis of the ABC triblock terpolymers towards the preparation of the respective gels, including investigations of crosslinking efficiencies and probing the stability besides morphological studies. Furthermore, the functionality, i.e. the ionic conductivity, of the gels should be precisely understood with regard to the diffusion of ions, to evaluate the self-healing progress in the end. Finally, the gel electrolytes will be used in test batteries as proof-of-principle for new electrolyte materials.
-
Research Unit (FOR) 5301 „FuncHeal“, Project P3: Reversible photoactive materials for flexible bulk-heterojunction solar cells
Prof. Dr. Kalina Peneva, Dr. Martin D. Hager
Funded by the German Research Foundation
Funding period: 2022 to 2026Organic solar cells have been studied intensively in recent years and the achievable efficiencies have been significantly increased. However, the long-term stability of these systems is still often a challenge. In addition to the photo-oxidation of the active materials, e.g., conjugated polymers, cracks in the active material or delamination of the active layer from the electrodes also play a major role, in particular for flexible solar cells. In this context, this project of the research unit FuncHeal investigates new concepts for self-healing of photoactive materials that are suitable for organic solar cells.
Two damage scenarios will be investigated: i) Mechanical damage in the active layer (donor-acceptor blend) and ii) damage through photooxidation. Through the design and synthesis of tailor-made donor and acceptor materials (from small molecules to polymers), the introduction of flexible groups or reversible groups is intended to achieve mobility of the material on the one hand (healing of cracks) and on the other hand the introduction of reversible groups to enable the exchange of chromophores (healing after photooxidation). These processes will be examined in detail in the pure donor materials as well as in the blend with appropriately adapted acceptor materials.
-
Research Unit (FOR) 5301 „FuncHeal“, Project P4: Stress and strain resisting polymer solar cells: Towards high flexibility
PD Dr. Harald Hoppe, PD Dr. Martin Presselt
Funded by the German Research Foundation
Funding period: 2022 to 2026The stability of polymer solar cells, in which at least one component of the photoactive layer is a conjugated polymer, is often studied as a function of the illumination spectrum, temperature (cycles), humidity, and influence of reactants such as oxygen or water. In addition, attention is paid to long-term stable mixing of electron donors and acceptors, which is referred to the morphological stability.
In particular for potential real applications, the flexible properties of organic solar modules are important, which, however, places increased demands on their mechanical stability. Due to the bending of solar modules that occurs during application, cracks in the active layer as well as detachment of the active layer from the charge extraction layers (or electrodes) can occur. The latter process is called delamination and it occurs when the adhesion between two layers is unsatisfactory, while cracks within a layer indicate insufficient cohesion. P4 is, therefore, dedicated to the prevention of cracks and delamination defects, as well as to their healing. The material systems are based on the donor / acceptor materials provided by a different project or on classical poly(3-alkylthiophenes) in combination with fullerene derivatives and later also non-fullerene acceptors. The photoactive layer also consists of at least ternary mixtures to be able to map the required properties. To investigate healing, films and solar cells on flexible substrates are stretched or bent, respectively, to provoke cracking and delamination. The defects created by the mechanical stress are characterized and quantified by microscopic and spectroscopic methods. After the healing, an analogous procedure is performed enabling a quantification of the healing.
-
Research Unit (FOR) 5301 „FuncHeal“, Project P5: Computational modeling of degradation and recovery processes of self-healing functional materials
Prof. Dr. Stefanie Gräfe
Funded by the German Research Foundation
Funding period: 2022 to 2026Self-healing materials are very interesting for many applications due to their ability to repair structural damage and, thus, to regain their mechanical stability and properties. For self-healing functional materials, in addition to the structural repair, ideally the functionality should also be recovered. The design of such materials is very challenging and can benefit from computational studies of the self-healing process and of the functionality down to the molecular level.
In this context, a large toolset of theoretical and simulation methods will be applied in this project to understand the degradation and recovery of various self-healing functional materials. The employed methods cover a range of relevant spatial and temporal scales: From larger-scale dynamical processes and structural properties (molecular dynamics and molecular mechanics calculations) to the molecular level (quantum chemical and hybrid quantum chemical-molecular mechanical calculations). The systems of investigation are chosen in close collaboration with the experimental projects of this research unit.
The overall objective of the project is the modelling and exploring the underlying mechanisms leading to degradation, thereby aiding the synthesis-oriented projects in identifying possibilities either to avoid degradation or to regenerate the material and the functionality. In particular, spectroscopic observables will be simulated such as UV/vis, Raman or 2D-correlation spectra which are used in the experimental investigations of self-healing processes. Eventually, general structure-property relationships will be revealed for functional self-healing materials which can help aiding the design of those materials.
-
Research Unit (FOR) 5301 „FuncHeal“, Project P6: Optical (micro)spectroscopy for characterizing structure and electronic properties of self-healing materials for energy conversion and storage
Prof. Dr. Benjamin Dietzek-Ivanšić
Funded by the German Research Foundation
Funding period: 2022 to 2026Project 6 will characterize the molecular mechanism in self-healing materials for energy conversion and storage by means of multimodal frequency- and time-resolved optical spectroscopy. The experiments will provide insights into the complete lifecycle of a functional self-healing material from damage to healing. In doing so, the project will gain a detailed understanding of the molecular processes occurring during healing of functional materials For polymer-based batteries and supercapacitors as well as organic solar cells.
To this end, the project’s focus is threefold:
- Investigation of the molecular structure changes during degradation and self-healing via in-situ vibrational spectroscopy and vibrational spectroelectrochemistry in combination with 2D correlation analysis to derive structureproperty relationships with respect to the underlying reversible self-healing chemistry;
- Studying transport and chemical reactivity to provide a molecular view on the diffusion and thus self-healing processes of the self-healing functional materials researched within FuncHeal, by combining fluorescence and non-linear Raman microscopy. Here, the molecular view will be correlated with macroscopic healing studies to enable tuning the molecular components inside the materials to achieve the desired macroscopic properties and
- Characterizing excited-state processes in damaged and healed materials for energy conversion to validate the light-induced function-determining process in self-healing materials for polymer solar cells. Furthermore, the project strives towards utilizing the spectroscopic signature of optically excited polymer materials to generate metrics to follow the kinetics of material degradation and healing.
-
Novel In-Situ Electrolytes for Electrochemical Capacitors
Prof. Dr. Andrea Balducci
Funded by the German Research Foundation
Funding period: 2021 to 2024Electrical double layer capacitors (EDLCs) are nowadays considered among the most important energy storage devices. In these devices the energy is physically stored, through the formation of an electric double-layer at the interface between (polarized) electrodes and ions of the electrolyte Carbon-based materials are currently the state-ofthe- art electrode materials in EDLCs. These materials, however, are produced by means of multi-step syntheses associated with a high energy demand and an excessive accumulation of waste and byproducts. This is in sharp contrast with the core idea of sustainable energy production and storage. In this project we aim to design carbon syntheses in such a way that the formed by-products are not treated as waste products, but instead generate a salt suitable for the realization of EDLCs. Thus, the by-products contribute to the functionality of the desired final product and waste accumulating purification steps are by-passed completely. For that reason, we call this approach the ’in situ electrolyte‘ concept. Combining the expertise on porous carbon development of the Borchardt group with the expertise on electrolyte design of the Balducci group we target the following aspects: Firstly, understanding the underlying principles of in situ electrolyte concept by investigating different activation agents, super acids and precursor materials, secondly, to utilized this strategy for the realization of lithium and sodium-based electrolytes, thirdly advancing the concept to metal-ion capacitors and fourthly developing a 3rd generation in situ electrolyte concept, in which even the electrolyte solvent can be generated from the waste products of carbon syntheses.
-
Superkondensatorentwicklung in Richtung effektiver Materialien mit erhöhter Energiedichte
Prof. Dr. Andrea Balducci
Funded by the German Federal Ministry for Economic Affairs and Energy
Funding period: 2021 to 2024 -
Horizon 2020 "Widening Participation": FunGlass - Center of functional and surface-functionalized glasses
Prof. Dr. Lothar Wondraczek
Funded by the European Commission
Funding period: 2015 to 2024 -
Co-ordination of the Priority Programme „Polymer-based Batteries“ (SPP 2248)
Prof. Dr. Ulrich S. Schubert
Funded by the German Research Foundation
Funding period: 2020 to 2023Amongst the different energy storage systems/batteries, polymer-based batteries represent an emerging technology. They feature interesting properties like lightweight, printability, flexibility as well as charging within few minutes (down to even seconds). Such polymer-based batteries can be fabricated utilising organic materials without the requirement for other critical raw materials. The well-defined structure of organic/polymer materials offers reliable structure-property relationships, and, thus, a well controllable and tuneable electrochemical behaviour can be achieved. The Priority Programme aims at the elucidation of structure-property-relationships, the design and synthesis of novel active materials, which will result in polymer-based batteries with preferably high capacities and longer lifetime over many cycles.
-
SPP 2248 "Polymer-based Batteries", Project: Entwicklung von Polymerelektrolyten komplementär zu Modellsystemen für Batterien auf Polymerbasis
Prof. Dr. Ulrich S. Schubert
Funded by the German Research Foundation
Funding period: 2020 to 2023 -
SPP 2248 "Polymer-based Batteries", Project: Entwicklung neuer redoxaktiver Polymere auf Basis von Benzimidazol, Benzoxazol und Benzothiazol – ein kombinierter theoretischer und experimenteller Screening-Ansatz
Prof. Dr. Ulrich S. Schubert
Funded by the German Research Foundation
Funding period: 2020 to 2023 -
Innovation Center CEEC Jena
Funded by the Free State of Thuringia and the European Regional Development Fund (ERDF)
Duration: 2022 to 2023With regard to a sustainable energy supply, energy storage and converters are central components of a successful energy transition. Polymers are promising materials in this context as they allow energy storage (i.e. batteries) and converters (e.g. solar cells) with special properties (e.g. flexible, printable, light weight, low carbon footprint). As part of this project, the infrastructure of the CEEC Jena is to be further expanded with regard to these polymers.
-
SPP 2248 "Polymer-based Batteries", Project: Aufklärung von Degradationsmechanismen in Polymer-basierten Dual-Ionen-Batterien und Entwicklung von Strategien zur Leistungsoptimierung
Prof. Dr. Ulrich S. Schubert
Funded by the German Research Foundation
Funding period: 2020 to 2023 -
SPP 2248 "Polymer-based Batteries", Project: Entwicklung von Aktivmaterialien für organische Batterien basie-rend auf elektropolymerisierten Polymeren mit stabilen organischen Radikalen
Prof. Dr. Ulrich S. Schubert
Funded by the German Research Foundation
Funding period: 2020 to 2023 -
Opto-electronic processes in photovoltaic structures interacting with nanodiamonds
PD Dr. Harald Hoppe
Funded by the German Research Foundation
Funding period: 2020 to 2023This project will pursue the implementation of nanodiamonds (NDs) towards enhancement of properties (chemical, electrical, opto-electronic) of organic photovoltaic (PV) solar cells. Structures prepared with NDs will be either layers (e.g. via extending or replacing charge extraction layers such as PEDOT:PSS or ZnO) or bulk (ternary bulk heterojunction system of organic solar cells). NDs will vary in size (1-10 nm) and surface termination (O, H), all very well defined by preparation. The structures will be characterized by advanced scanning probe methods (KPFM, C-AFM) in the dark and under illumination (DUV-vis, sol. simulator) and macroscopic (e.g. current-voltage) characterizations. Physicochemical interactions between NDs and other charge transport or charge generation materials will be monitored spectroscopically (Raman scattering, FTIR, PL). It is expected, that the project will enhance the knowledge on the role and possible application of NDs in organic photovoltaics (light scattering, active photoresponse, optical and chemical interaction, charge extraction and transport) and which configuration (size and termination of NDs, layered or bulk, type of photovoltaic blend) will exhibit the best development output in terms of performance and stability (power conversion efficiency, optical and mechanical properties, degradation).
-
Horizon 2020 "PowerskinPLUS: Highly advanced modular integration of insulation, energising and storage systems for non-residential buildings" European Commission – Horizon 2020 Framework Program – Industrial Leadership
Prof. Dr. Lothar Wondraczek
Funded by the European Union
Funding period: 2019 to 2023 -
Co-ordination ETN POLYSTORAGE: European Training Network in innovative Polymers for next-generation electrochemical energy storage
Prof. Dr. Ulrich S. Schubert
Funded by the European Union
Funding period: 2019 to 2023 -
Horizon 2020 "enjulii: Entropic rejuvenation of thin glass products by liquid metal immersion" European Research Council (ERC) - Horizon 2020 Framework Program - Excellent Science
Prof. Dr. Lothar Wondraczek
Funded by the European Union
Funding period: 2021 to 2022 -
Assistance systems for intelligent cattle farming (CattleHub), experimental area „Sensors and energy supply”
PD Dr. Harald Hoppe
Funded by the Federal Ministry of Food and Agriculture (BMEL)
Funding period: 2019 to 2022By means of digital sensor technology, almost all vitality parameters of a cow or a bovine can be determined and monitored in real time today. These are, for example, body temperature, blood pressure, pulse and heart rate, blood oxygen saturation, motion parameters or the amount of feed and water intake. This allows irregularities in the daily routine to be detected, conclusions to be drawn about the health of the animals, or animal-specific decisions to be derived when managing the animals. What fitness trackers can do for humans today can thus be used in a very similar way for animal welfare.
In the “Sensors & Energy Supply” field of experimentation, such sensor systems are examined from the point of view of their general usability by farmers. Which sensor technology is suitable for use on animals and where are the limitations and boundaries? Which parameters can be collected with which sensor at which position and how often is this actually necessary? By which energy supply can the sensor technology be fed more persistently or sustainably? After all, those sensors require energy and an animal cannot be regularly plugged into a socket like a cell phone. In the end, of course, it all has to pay off financially, which makes the task correspondingly more difficult.
These and other questions are being experimentally investigated and scientifically evaluated not only for products that are currently commercially available on the market, but also for novel sensor, as well as energy generation, storage and saving technologies. The answers to be found will be processed during the course of project for both, research and practical users. The answers may also represent an important decision-making aid for the introduction of digitalization in animal-related agriculture.
-
CRC/TRR 234 CataLight, Project A01: Strategies for Molecular Repair and Self-regulation in Light-driven Catalysis for Hydrogen Evolution
Prof. Dr. Benjamin Dietzek
Funded by the German Research Foundation
Funding period: 2018 to 2022The project will strive for developing active repair strategies leading to recycling of the molecular catalyst and creating self-regulating supramolecular light harvesting complexes, which funnel excitation energy to the photocatalyst. Active repair by 1O2 has been demonstrated for the [(tbbpy)2Ru(tpphz)PtI2](PF6)2 system leading to a significantly increased overall TON. More selective and milder active repair mechanisms shall be developed utilizing, e.g. catalytic re-oxidation by polyoxometalates. Self-regulating antenna complexes will be incorporated based on hydrogen bonding and ππ-interactions, which disintegrate, e.g. upon changes of pH value.
-
CRC/TRR 234 CataLight, Project A03: Rylene Dyes as Photosensitizers and Antenna Systems
Prof. Dr. Kalina Peneva
Funded by the German Research Foundation
Funding period: 2018 to 2022The project focuses on the design of tailored rylene antennas and photosensitizers with broad visible light absorption. Chemical functionalization of the dye will enable coupling to catalytic units, allow soft matter integration, tune the redox potentials and adjust rylene solubility. Additionally, pH-responsive chromophores will be prepared for activity regulation and as local pH probes. Photophysical studies will provide a deeper understanding of the electron transfer processes as well as deactivation and degradation pathways to identify general design guidelines for novel rylene photosensitizers for oxidative and reductive light-driven chemistry.
-
CRC/TRR 234 CataLight, Project B01: Structure-Property Relationships of Functional Copolymers on DNA Nanosheets
Prof. Dr. Ulrich S. Schubert
Funded by the German Research Foundation
Funding period: 2018 to 2022DNA origamis will be used for the precise spatial positioning of functional moieties such as molecular compounds (e.g. dyes, photosensitizers, catalysts and electron relays) as well as functional copolymers. We will elucidate structure-property relationships in these assemblies and study energy/electron transfer processes. Precise assembly of two molecular entities on the surface, assembly of molecular moieties next to copolymers, two functional copolymers, which are assembled in close proximity as well as the surface-routing of single functional moieties is developed.
-
CRC/TRR 234 CataLight, Project B02: Integration of Photoredox-active Complexes in Redox-active Polymers for Light-induced Charging and Discharging by Additional Integrated Molecular Catalysts
Prof. Dr. Ulrich S. Schubert
Funded by the German Research Foundation
Funding period: 2018 to 2022The project aims at the integration of photoredox-active ruthenium photosensitizers into redox-active copolymers by supramolecular interactions, e.g. ionic interactions or π-stacking. Redox-active moieties such as anthra¬chinones and viologens will be embedded within the polymer and can act as multi-electron storage components to accept electrons transferred by the photosensitizer. Further functionalization of the polymer by metal complex HER catalysts will be used to harvest the stored electron for subsequent catalytic turnover. The influence of the copolymer structure on the electron transfer between photosensitizer – electron relay – catalyst will be investigated in detail.
-
CRC/TRR 234 CataLight, Project B03: “POMbranes” – Incorporation of Catalytically Active Polyoxometalates into Integral Asymmetric Block Copolymer Membranes
Prof. Dr. Felix H. Schacher
Funded by the German Research Foundation
Funding period: 2018 to 2022The project will prepare hierarchically structured block copolymer membranes functionalized with molecular PS and POM catalysts for light-driven WOC and HER. Immobilization by electrostatic, supramolecular and covalent anchoring will be developed and the consequences for light-driven catalytic reactivity and component stability will be explored. For covalent anchoring, suitable organo-functionalization of the POMs will be developed together with complementary functionalization of the block copolymer. Synergistic reaction enhancement by membrane-catalyst interactions will be explored together with the catalytic performance of the composite membrane under flow.
-
CRC/TRR 234 CataLight, Project B05: Self-regulating Photocatalytic Materials Based on pH-responsive Block Copolymers
Prof. Dr. Felix H. Schacher
Funded by the German Research Foundation
Funding period: 2018 to 2022The project develops photocatalytic materials where activity or accessibility of a catalytic center is controlled by external parameters such as pH value or temperature. This will ultimately lead to composites which provide optimum reaction conditions for prolonged periods. We will co-integrate Ru- or rylene-based photosensitizers and Pt- or Co-based catalysts into colloidal polymer models where changing environmental conditions lead to modification of swelling degree or charge density. These structural transitions will be described by theoretical modeling.
-
CRC/TRR 234 CataLight, Project B06: Molecular Functionalization of Carbon Nitride Polymers for Light-driven Water Splitting
Prof. Dr. Benjamin Dietzek
Funded by the German Research Foundation
Funding period: 2018 to 2022This project develops a fundamental mechanistic understanding of the interaction of polymeric carbon nitride (CNx)-based materials with molecular redox catalysts and molecular photosensitizers. The project will focus on elucidating factors governing the dynamics of charge separation, charge recombination and catalytic turnover in HER and WOC catalysis. The interdisciplinary approach will yield design rules for the development of molecularly functionalized photoactive CNx materials, and advance our understanding of the fundamental advantages and bottlenecks of CNx-based systems for light-driven oxidative and reductive chemistry.
-
CRC/TRR 234 CataLight, Project B07: Carbon Nanomembranes as Asymmetric, Two-dimensional Matrices for the Immobilization and Dynamic Covalent Regeneration of Molecular Photosensitizers and Hydrogen Evolution Catalysts
Prof. Dr. Andrey Turchanin
Funded by the German Research Foundation
Funding period: 2018 to 2022The project aims at the development of photocatalytic materials consisting of carbon nanomembranes (CNMs), molecular photosensitizers (PSs) and molecular, cobalt-based catalysts (CAT) for HER catalysis. This includes studies of previously unexplored, photocatalytically relevant properties of CNMs, the preparation and investigation of proof of principle photocatalytic devices, in which PS and CAT are covalently immobilized on the CNM, and exploration of the regeneration of inactive CNM components by means of dynamic covalent exchange reactions.
-
CRC/TRR 234 CataLight, Project C01: Spatially and Temporally Resolved Spectroelectrochemistry
Prof. Dr. Benjamin Dietzek
Funded by the German Research Foundation
Funding period: 2018 to 2022The project will develop nanoscale and ultrafast time-resolved spectroelectrochemistry to study soft matter integrated molecular catalysts. Environmental effects on the structural features and excited-state properties of reduced/oxidized molecular species when embedded into a soft matter matrix will be deciphered. The project combines electrochemical methods with (i) tip-enhanced spectroscopies and (ii) ultrafast pump-probe transient absorption spectroscopy to study the reactivity of matrix-embedded photosensitizers and catalysts. Finally, ultrafast near-field transient absorption spectroscopy will be applied to photocatalytically active films.
-
CRC/TRR 234 CataLight, Project C05: Structures and Processes in the Photocatalytic Hydrogen Evolution by Immobilized Metal Complexes Studied from First Principles
Prof. Dr. Stefanie Gräfe
Funded by the German Research Foundation
Funding period: 2018 to 2022The project will perform theoretical calculations to explore the consequences of photosensitizer-catalyst dyad integration into soft matter matrices. To understand the performance of model Ruthenium-photosensitizer – bridging ligand – Platinum catalyst dyads for HER, we will initially explore their embedding and photophysical properties on NiO as model surface electrode. Subsequently, we will study more complex soft matter matrices to elucidate how charge separation, charge recombination or interfacial charge transfer affect the catalytic reactivity observed.
-
EDLstruct: Effect of carbon electrodes porous structure and electrolyte properties on the formation and composition of the electrical double-layer
Prof. Dr. Andrea Balducci
Funded by the German Research Foundation (DFG)
Funding period: 2018 to 2022The formation of an electrical double-layer (EDL) in a porous conductive electrode, e.g., from activated carbon (AC), represents one of the most appealing strategies for fast storage of energy and, consequently, for the realization of high power energy storage devices. However, the existing models of EDL which consider the electrolyte border with a flat surface cannot be applied to the case of microporous (<2 nm) AC electrodes. Recently, in-situ techniques have provided important information about the EDL properties in AC electrodes, yet they were implemented with a unique electrolyte and a unique type of porous carbon. Therefore, the objective of the EDLstruct project is to implement a variety of organic electrolytes with a variety of carbons of well-defined porous structure in order to study: i) the influence of pore size and molecules size on the ions/solvent ratio under polarization, and ii) to find correlations between the EDL structure and the decay of electrochemical performance of carbon electrodes depending on temperature and electrodes potential. In order to realize this program, strong competence in carbonaceous materials (PUT group) as well as in electrolytes (FSU Jena group) is needed. In addition, the use of in-operando techniques (PUT) is required to monitor the effects of molecular fluxes and EDL decomposition during polarization of electrodes. Hence, the research objectives will be reached by conducting 5 work packages. FSU Jena will design a number of non-aqueous electrolytes (WP1), while PUT will design and characterize ACs with different structural properties (WP2). The electrochemical performance of AC electrodes in combination with the considered electrolytes will be investigated by both groups. In order to acquire information about the double-layer formation, post-mortem analyses of polarized electrodes by thermoprogrammed desorption and in-operando electrical dilatometry will be carried out by PUT (WP3). The influence of the electrode-electrolyte interaction on the thermal stability of the double-layer will be investigated in WP4 by FSU Jena (TGA/DSC) and PUT (electrochemical on-line mass spectrometry). The experimental results will be critically discussed in WP5, giving rise to publications and presentations at international conferences.
-
Redox-active ionic liquids in redox-flow-batteries
Prof. Dr. Ulrich S. Schubert
Funded by the German Research Foundation
Funding period: 2018 to 2022Redox flow batteries (RFBs) are interesting candidates for stationary energy storage. Their capacity and power can be scaled independently by adjusting the size of the storage tank and the cell stack, respectively. In contrast to classical batteries, redox flow batteries (RFBs) are based on active materials, which are dissolved (suspended) in a liquid electrolyte. Consequently, the achievable energy densities are limited due to the presence of a non-active solvent (often water). The most investigated RFB is the all vanadium RFB, which utilizes vanadium ions dissolved in sulfuric acid. In recent years, the interest in organic (polymeric) active materials is steadily growing. This joined proposal aims to develop redox-active ionic liquids (IL) as new materials for the electrolytes of RFBs. All molecules present in the electrolyte will therefore be redox-active. Additionally, the limited voltage window of aqueous electrolytes will be extended. Consequently, higher energy densities will be achievable.The project will be investigated by the two partners at the Friedrich Schiller University Jena (Institute for Technical Chemistry and Environmental Chemistry as well as Institute for Organic Chemistry and Macromolecular Chemistry). Latter partner will contribute to the synthesis and structural characterization of novel redox-active ionic liquids. These ILs will comprise redox-active moieties and ionic groups. The properties will be tuned by the respective structure of the IL. Furthermore IOMC will perform the tests of the synthesized materials in test RFB cells. The ITUC will contribute to the extensive characterization of the synthesized materials. In particular the electrochemical as well as thermal properties will be of interest.
-
Block copolymer based hybrid materials for direct electrochemical biosensing of nucleic acids and hemoproteins
Prof. Dr. Felix Helmut Schacher
Funded by the German Research Foundation (DFG)
Funding period: 2018 to 2022
Project partner: Dr. Larisa Sigolaeva, Moscow State University, RussiaThe project aims at the development of experimentally available reagentless electrochemical methods for identification and quantification of nucleic acids and hemoproteins. The level of circulating tumor DNA, RNA, or micro RNA can be taken as a measure for diagnostic and prognostic approaches to different types of cancer. Simultaneously, a considerable release of hemoprotein cytochrome c occurs during the treatment of cancer cells with anti-cancer drugs, and this level can then be taken as measure for the efficiency of a certain drug, the screening of new potential drug candidates or, in turn, as an indicator for cell resistance towards anti-cancer therapy. Simultaneous detection and quantification of both these biomarkers could considerably increase the efficiency of anti-cancer therapy with respect to (subtle) differences between different patients and help in developing strategies for personalized medicine.The sensitivity of direct electrochemical analysis strictly depends on the degree of integration of the analyte within the working electrode, in our case a nanocomposite material. For this purpose, a combination of carbon nanomaterials (carbon nanotubes or graphene oxide), noble metal nanoparticles (gold or silver nanoparticles), and amphiphilic block copolymers will be used. Therefore, we first focus on principles of organic/inorganic nanocomposite fabrication based on uniformly solubilized carbon nanomaterials and, eventually, noble metal nanoparticles. The employed amphiphilic diblock copolymers will serve a triple role. First, they are used as dispersing agent for the carbon nanomaterial due to hydrophobic interactions between the latter and the hydrophobic block. Further, the hydrophilic (and ionic) part of the material can be used to complex metal ions, followed by reduction to metal nanoparticles. Finally, after deposition on suitable substrates the diblock copolymer serves as matrix material for specific interaction with target biomolecules. The role of the carbon nanomaterial is to improve conductivity of the entire composite and the efficiency of electron transfer. Finally, the presence of metal nanoparticles is expected to catalyze electrochemical reactions. In total, these effects will synergistically provide a considerable improvement of biosensor sensitivity and selectivity for direct electrochemical analysis of both nucleic acids and cytochrome c.During the course of this project, a thorough examination of bioanalytical properties will involve a systematic investigation towards optimal construction and composition of the block copolymer/carbon nanomaterial/metal nanoparticle hybrid material. Subsequently, best working constructs will be used to assess biosensor characteristics, followed by the development of application strategies for direct electrochemical analysis of real samples (whole blood, plasma or serum).
-
Horizon 2020 "Excellent Science" (ERC) : Unifying concepts in the topological design of disordered solids - UTOPES
Prof. Dr. Lothar Wondraczek
Funded by the European Commission
Funding period: 2016 to 2022Glasses have traditionally been enabling materials to major societal challenges. Significant breakthroughs on many areas of technological progress have been very closely linked to the exploitation of glassy materials. It is strong consensus that this key role will persist in the emerging solutions to major global challenges in living, energy, health, transport and information processing, provided that the fundamental limitations of the presently available empirical or semi-empirical approaches to glass processing can be overcome.
In the coming decade, it is therefore a major task to take the step towards ab initio exploitation of disordered materials through highly-adapted processing strategies. This requires pioneering work and in-depth conceptual developments which combine compositional design, structural evolution and the thermo-kinetics of material deposition into holistic tools. Only those would significantly contribute to solving some of the most urgent materials needs for glass applications in functional devices, be it in the form of thin films, particles or bulk materials.
UTOPES challenges today’s engineering concepts towards the conception of such tools. For that, melt deposition, isothermal deposition from liquid phases, and gas-phase deposition of non-crystalline materials will be treated – within the class of inorganic glasses – in a generalist approach, unified by the understanding that glass formation represents the only strict deviation from self-organization, and that, hence, the evolution of structural complexity in glassy materials can be tailored on any length-scale through adequate processing. Providing a topological scheme for the quantification and chemical tailoring of structural complexity, UTOPES will answer to the challenge of finding order in disorder, and will thus break the grounds for the third generation of glasses with properties beyond what is presently thought as the limits of physical engineering.
-
Innovation Center CEEC Jena
Funded by the Free State of Thuringia and the European Union funds under the European Regional Development Fund (ERDF)
Funding period: 2017 to 2022The CEEC Jena Innovation Center offers research and development services as well as education and training in three areas – light-energy conversion, energy storage and clean tech. The grant application concerned the current activities and characteristics of the CEEC Jena in the field of energy storage.
Innovative energy storage technologies are an important element for the success of the energy transition in Germany and for future value creation as an industrial nation in a large number of product areas. The CEEC Jena is specialized in developing next-generation batteries ("Beyond Lithium Batteries") in a holistic research approach that ranges from application-oriented basic research to prototyping. In contrast to competing approaches, the research at the CEEC Jena aims at the replacement of metals (for example, cobalt in lithium batteries or rare earths in nickel-metal hybrid batteries) by environmentally friendly alternatives made of polymers (plastics) or ceramics.
The scientific, economic and social potential of innovative battery systems – from small printable polymer batteries to sodium ion batteries and large stationary energy storage devices (for example, polymer redox flow batteries) – has been confirmed by numerous studies by renowned experts. The CEEC Jena was able to successfully establish itself in this segment and has a clear development concept in order to exploit the opportunities offered by the energy transition for the region. The CEEC Jena thus supports the objectives defined in the framework of the Regional Research and Innovation Strategy (RIS 3 Thuringia). On the one hand, the goal is to build up and expand the scientific top positions and, at the same time, create the conditions to build up complete production and value chains for battery systems in Thuringia and Germany.
-
Sliding contacts in liquid environments: A nanotribology study
Prof. Dr. Enrico Gnecco
Funded by the German Research FoundationUnderstanding the influence of the surrounding environment on the sliding friction between two solid surfaces in liquids is of utmost importance for applications to MEMS/NEMS or colloidal systems as well as to shed light on the dynamics of formation and rupture of single contact junctions. In this project we aim to perform an experimental investigation of the mechanical properties of contacts formed by sharp silicon tips on sodium chloride, silica, calcite, and molybdenum disulfide using friction force microscopy. The sample surfaces will be exposed to electrolite acqueous solutions to explore specific-ion effects all along the Hofmeister series, and to polar and nonpolar solvents (ethanol, acetone, dichlorobenzene, etc.) with varying viscosities. The different interaction potentials will be quantitatively reconstructed from the time variations of the friction forces acting on the tip with an original method proposed by one of the applicants (RB) and recently applied to reconstruct the free energy landscape of protein unfolding under mechanical load. The results so obtained will be compared to complementary information available with dynamics AFM techniques.
-
LIBRA - Low cost battery based on abundant elements
Prof. Dr. Philipp Adelhelm
Funded by the Federal Ministry of Education and Research (BMBF) and the the European Union
Project partners: Prof. Dr. Teofilo Rojo (CIC EnergiGUNE, Álava, Spain), Prof. Dr. Shinichi Komaba (Tokyo University of Science, Tokio, Japan)
Funding period: 2018 to 2021 -
Redox-active ionic liquids in redox-flow batteries
Prof. Dr. Andrea Balducci/Prof. Dr. Ulrich S. Schubert
Funded by the German Research Foundation (DFG)
Funding period: from 2018Redox flow batteries (RFBs) are interesting candidates for stationary energy storage. Their capacity and power can be scaled independently by adjusting the size of the storage tank and the cell stack, respectively. In contrast to classical batteries, redox flow batteries (RFBs) are based on active materials, which are dissolved (suspended) in a liquid electrolyte. Consequently, the achievable energy densities are limited due to the presence of a non-active solvent (often water). The most investigated RFB is the all vanadium RFB, which utilizes vanadium ions dissolved in sulfuric acid. In recent years, the interest in organic (polymeric) active materials is steadily growing. This joined proposal aims to develop redox-active ionic liquids (IL) as new materials for the electrolytes of RFBs. All molecules present in the electrolyte will therefore be redox-active. Additionally, the limited voltage window of aqueous electrolytes will be extended. Consequently, higher energy densities will be achievable.The project will be investigated by the two partners at the Friedrich Schiller University Jena (Institute for Technical Chemistry and Environmental Chemistry as well as Institute for Organic Chemistry and Macromolecular Chemistry). Latter partner will contribute to the synthesis and structural characterization of novel redox-active ionic liquids. These ILs will comprise redox-active moieties and ionic groups. The properties will be tuned by the respective structure of the IL. Furthermore IOMC will perform the tests of the synthesized materials in test RFB cells. The ITUC will contribute to the extensive characterization of the synthesized materials. In particular the electrochemical as well as thermal properties will be of interest.
-
The combined use of computational screening and electrochemical characterization for the identification of new electrolyte components for supercapacitors
Prof. Dr. Andrea Balducci
Funded by the German Research Foundation (DFG)
Funding period: 2017 to 2020The project proposes the use of an innovative strategy for the identification of new electrolyte components for electrochemical double layer capacitors (EDLCs), in which computational screening and electrochemical characterization are combined. In order to realize advanced EDLCs the introduction of new electrolyte components, solvents and salts, is strongly needed. To identify a new component experimentally is difficult and time consuming, especially when dealing with a completely new group of compounds. In order to avoid a time consuming 'trial and error' approach, the introduction of an effective 'virtual' (i.e. computer-based) screening method is therefore of great importance. Such a screening should allow for a fast prediction of the properties of a large number of compounds from many different classes, which in turn would enable the identification of the most promising candidates. Recent work showed that this innovative approach can be successfully used for the identification of new electrolyte components and, thus, it should be seen as a new and powerful tool for the development of advanced EDLCs. Nevertheless, further investigations are needed to optimize such a strategy and, also, to understand the chemical-physical and electrochemical properties of new solvents and new conducting salts suitable for the EDLC technology.
-
PhotoFlow - Photoelectrochemical Redox-Flow batteries
Prof. Dr. Ulrich S. Schubert
Funded by the German Federal Ministry for Economic Affairs and Energy (BMWI)
Funding period: 2017 to 2020 -
Towards Photoactive Membranes for Artificial Photosynthesis
Prof. Dr. Andrey Turchanin, Prof. Dr. Benjamin Dietzek, Prof. Dr. Ulrich S. Schubert
Funded by the German Research Foundation (DFG)
Funding period: 2017 to 2020 -
Sodium-ion storage in carbon nanomaterials
Prof. Dr. Philipp Adelhelm
Funded by the German Research Foundation (DFG) and the Sino-German Center for Research Promotion (SGC)
International joint project with the National Center for Nanoscience and Technology, Peking (China)
Funding period: 2017 to 2020The search for efficient, reliable and low-cost electrochemical energy stores is an on-going and societally highly relevant task. As lithium-ion battery (LIB) technology more and more approaches its intrinsic limits, a range of alternatives is currently being studied. This also includes sodium-ion batteries (NIBs) that are largely motivated by the abundance of sodium. Even without sodium’s abundance, there is a clear and general need to develop potential alternatives to conventional LIB technology as a massive increase in materials supply is expected once electrification of transport and stationary energy storage enter the mass market. Using carbon materials in NIBs is therefore a straightforward approach to render batteries based on abundant elements. The use of carbon materials in NIBs is, however, poorly understood and so far does not meet all relevant criteria for application (capacity, kinetics, coulombic efficiency, redox potential). Overall, there is a clear need for more fundamental understanding of storing sodium-ions in carbon structure. Here, the project aims at making some important contributions. Carbon materials with tailored structure and chemical composition will be synthesized and their ion storage behavior in sodium (and lithium) cells will be evaluated with the aim to provide insights into the complex storage phenomena as well as the underlying physical-chemical principles. As a main content of the project, the influence of surface chemistry, morphology and hetero atom doping on the kinetics and thermodynamics of the charge storage mechanism as well as on the surface film formation (solid electrolyte interphase, SEI) will be studied. This way, we aim at overcoming current limitations for carbon materials for sodium-ion batteries and will also be able to judge on whether carbon nanomaterials remain model systems or can really contribute to the development of practical NIBs.
-
Tailored Optical Fibres, TP1.3 &4.1
Prof. Dr. Lothar Wondraczek
Funded by the Federal Ministry of Education and Research (BMBF)
Funding period: 2017 to 2019 -
SPP 1594 "Topological Engineering of Ultra-Strong Glasses"
Prof. Dr. Lothar Wondraczek (Co-ordinator)
Funded by the German Research Foundation (DFG)
Funding period: 2012 to 2019Accepting brittleness and benefiting from their optical properties and universal processability, glassy materials have found their traditional applications in environments with low levels of tensile stress. It was recognised, however, that intrinsically glasses represent the strongest man-made material, which can be produced on large-scale. Only for low resistance to surface damage, the uniquely high levels of intrinsic strength can presently not be made use of. In a broader context, covering all classes of disordered materials (distinguished by the nature of chemical bonds), brittleness, stiffness, elastic limit and the practical absence of ductility originate from their molecular, mid-range and surface topology. In inorganic as well as metallic glasses and in contrast to any other solid material, mechanical properties must be considered in a twofold way: on the basis of structural length scales and dynamics. Identification of determinant topological and density constraints and their engineering towards ultrahigh toughness is now seen as the major future breakthrough of the field. As to yet disregarded synergy arises from the joint treatment of both classes of glass: from a topo-mechanical view, both materials appear to follow the same constitutive principles of coordination, packing density, free volume, structural dynamics, structural heterogeneity and extreme mid- to long-range homogeneity. The Priority Programme has the objective to condense existing experimental and theoretical competencies within Germany to enable a conceptual breakthrough in the understanding and design of the mechanical properties of glasses. For the first time, inorganic and metallic glasses will be considered in a joint context. Key to topical success, international visibly and long-lasting impact is the effective coordination of this effort for which a number of central activities are planned, including the organisation of national and international workshops and conferences, the promotion of young researchers, equal opportunity actions, setting-up a mentoring programme and ensuring high-impact outreach and networking.
-
Benzotriazinyl radical containing polymers as bipolar active electrode material in organic secondary batteries
Prof. Dr. Ulrich S. Schubert
Funded by the German Research Foundation (DFG)
Funding period: 2016 to 2019The growing interest in mobile devices and their increasing integration into the World Wide Web (Internet of Things) necessitates the availability and development of small and flexible energy-storage systems. However, lithium ion batteries, which represent the current benchmark technology, do not allow the assembly of flexible batteries and demand environmentally questionable techniques for production and processing of raw materials as well as for the final disposal of disused devices. Hence, current research focuses more and more on thin film batteries that are based on organic redox active molecules, which are prepared through organic synthesis and disposed free of residues via burning. Furthermore, their (electro)chemical properties can be easily tuned through choosing a suitable chemical structure. Unfortunately, small molecules tend to dissolve in the used electrolyte leading to a significantly decreased lifetime of the battery. Thus, the monomeric redox active units are integrated into long chain polymers, which possess a substantially decreased solubility.In the course of the project, in particular polymers that are based on the benzo-1,2,4-triazinyl radical are developed. This molecule features electrochemical reversibility, high stability against air and moisture, as well as facile synthetic accessibility. Its redox characteristics can be, furthermore, easily tuned through the molecular substitution pattern, which enables even the preparation of systems that can serve both as anode and cathode material, allowing the construction of bipolar, i. e. poleless, batteries.The increase of the batteries still low theoretical capacity (from around 60 to over 100 mAh g-1) represents a central goal, which should be mainly achieved through the reduction of the molar mass of the monomer. (Electro)chemical stability, cell voltages of around 1.2 V (to allow for aqueous electrolytes), and polymerizability of the molecule have to be maintained, which is ensured through the investigation of structure-property relationships in the course of substituent variations to enable a systematic optimization of the system. The characterization process comprises the preliminary characterization of the monomeric and polymeric compounds in solution and in the solid state, the processing of the polymers with conductive additives and binder materials toward thin film composite electrodes and their subsequent characterization, as well as the assembly and comprehensive investigation of half-organic (using lithium or sodium as anode material) and full organic cells. Furthermore, the preparation of the batteries is optimized, in particular with regard to the used additives and the thin-film processing (doctor blading, inkjet printing).
-
Thermodynamic and kinetic properties of conversion reactions in new, sodium based battery systems
Prof. Dr. Philipp Adelhelm
Funded by the German Research Foundation (DFG)
Funding period: 2016 to 2019The development of safe and low cost electrochemical energy storage devices with high energy densities is one of the key challenges for current battery research. Current lithium-ion battery technology is mostly based on the reversible formation of intercalation compounds with layered structure. Higher capacities can be achieved by compounds that undergo so-called conversion reactions with lithium. The general reaction can be written as MaXb + (bc)Li = aM + b LicX with M being a transition metal (Fe, Co, Ni, Cu, etc.), X being a non-metal (F, O, N, P, S, etc.), and Li being lithium. Despite the intensive research in this field, no suitable electrode material could be identified so far that exhibits suitable cell characteristics for application at room temperature. Aim of the present research proposal is to investigate the largely unknown cell chemistry of sodium based conversion reactions for their use in room temperature sodium-ion batteries. The second important aim is to compare the cell chemistry of sodium based conversion reactions with the analogue lithium system. Similarities and differences between both reactions will be used to deepen the fundamental understanding of the underlying mechanisms of conversion reactions. As a result, general guidelines for the improvement of conversion systems towards application might be formulated. In both cases, experiments using thin film model electrodes allow the most accurate and exact investigations. Here, an important advantage of sodium over lithium is the easier analytical access due to the higher atomic weight of Na compared to Li. Objective of the subproject bulk phase is the systematic investigation of the general cell chemistry of conversion reactions and their structural characterization with standard methods (XRD, SEM, TEM, XPS, galvanostatic cycling, cyclic voltammetry, mass spectrometry). Important aim of the subproject is, to identify the most promising compounds MaXb for the comparison between sodium and the analogue lithium systems. Objective of the subproject model systems is the preparation of highly defined model electrodes and cells from selected compounds by means of pulsed laser deposition with the aim to develop suitable in situ experiments.
-
Redox chemistry of ternary graphite intercalation compounds: Theory and Experiment
Prof. Dr. Philipp Adelhelm
Funded by the German Research Foundation (DFG)
Project partner: Prof. Dr. Doreen Mollenhauer (Justus-Liebig-Universität Gießen)
Funding period: 2017 to 2018 -
Gas- and liquid-phase deposition of non-crystalline solids
Prof. Dr. Lothar Wondraczek
Funded by the Carl-Zeiss-Stiftung
Funding period: 2015 to 2019 -
HORIZON 2020 Flagship Graphene-based disruptive technologies
Prof. Dr. Andrey Turchanin
Funded by the European Commission
Funding period: 2016 bis 2018 -
Protic ionic liquids as innovative electrolytes for lithium-ion batteries
Prof. Dr. Andrea Balducci
Funded by the German Research Foundation (DFG)
Funding period: 2015 bis 2018 -
Self-Healing Inspired by Nature: Exocytosis-Like Repair of Membranes and Interfaces Composed of Self-Assembled Luminescent Dyes
part of the SPP1568 Design and Generic Principles of Self-healing Materials
PD Dr. Martin Presselt
Funded by the German Research Foundation (DFG)
Funding period:2014 - 2018 -
Horizon 2020 "Industrial Leadership": Large Area Fluidic Windows
Prof. Dr. Lothar Wondraczek (Co-ordinator)
Funded by the European Commission
Funding period: 2015 bis 2017 -
Self-healing block copolymer films from mechanistic understanding towards applications in coatings and membranes
Prof. Dr. Felix Helmut Schacher
Funded by the German Research Foundation (DFG)
Funding period: 2014 bis 2017
DFG project in the SPP1568 -
Self-organized dye-nanostructures for photovoltaic applications
PD Dr. Martin Presselt
Funded by the Federal Ministry of Education and Research (BMBF)
Funding period: 2013 - 2017